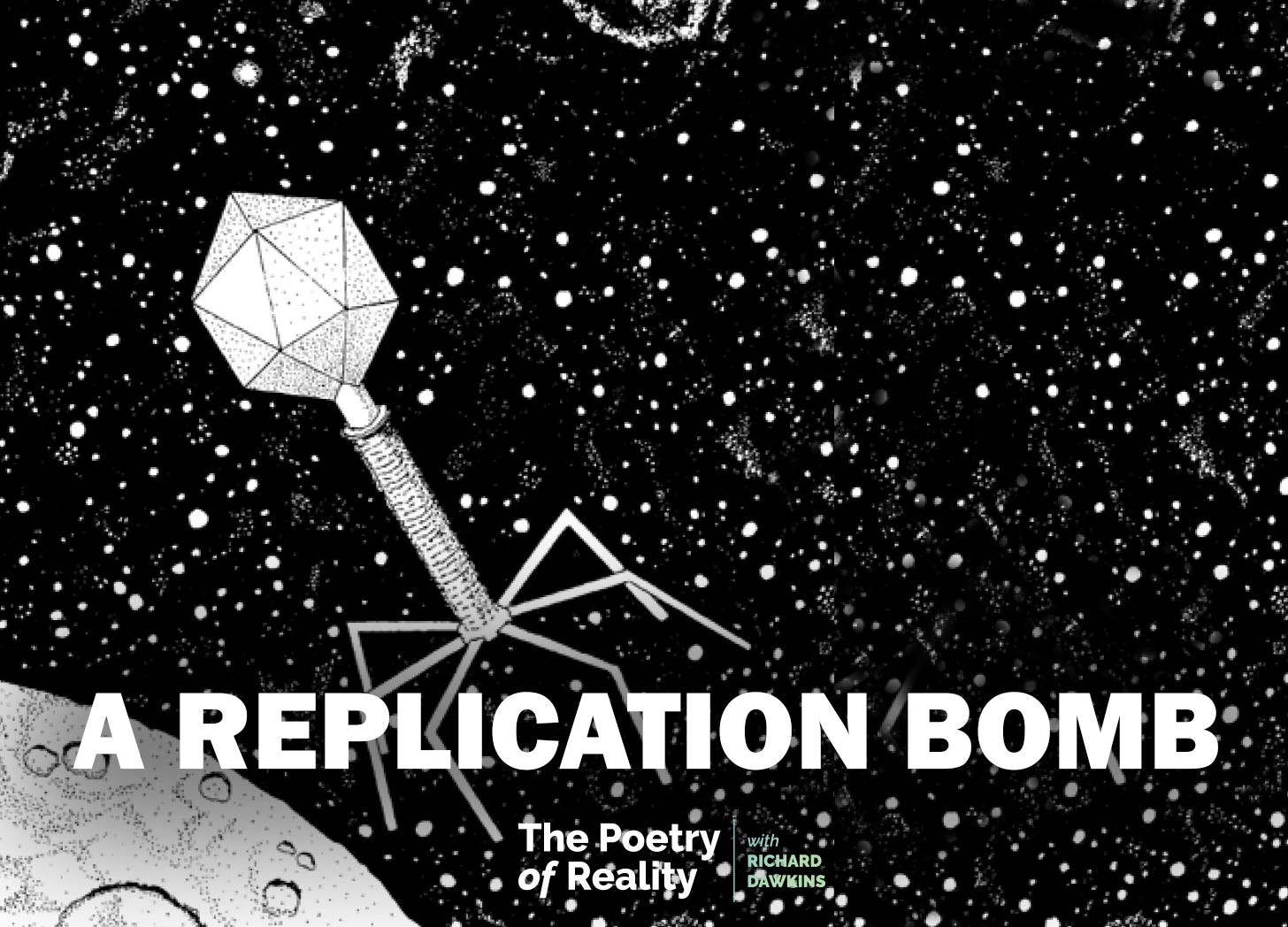
Most stars—and our sun is typical—burn in a stable manner for thousands of millions of years. Very rarely, somewhere in the galaxy a star suddenly flares without obvious warning into a supernova. Within a period of a few weeks, it increases in brightness by a factor of many billions and then dies away to a dark remnant of its former self. During its few high days as a supernova, a star may radiate more energy than in all its previous hundred million years as an ordinary star. If our own sun were to “go supernova,” the entire solar system would be vaporized on the instant. Fortunately this is very unlikely. In our galaxy of a hundred billion stars, only three supernovas have been recorded by astronomers: in 1054, in 1572, and in 1604. The Crab Nebula is the remains of the event of 1054, recorded by Chinese astronomers. (When I say the event “of 1054” I mean, of course, the event of which news reached Earth in 1054. The event itself took place six thousand years earlier. The wave-front of light from it hit us in 1054.) Since 1604, the only supernovas that have been seen have been in other galaxies.
There is another type of explosion a star can sustain. Instead of “going supernova” it “goes information.” The explosion begins more slowly than a supernova and takes incomparably longer to build up. We can call it an information bomb or, for reasons that will become apparent, a replication bomb. For the first few billion years of its build-up, you could detect a replication bomb only if you were in the immediate vicinity. Eventually, subtle manifestations of the explosion begin to leak away into more distant regions of space and it becomes, at least potentially, detectable from a long way away. We do not know how this kind of explosion ends. Presumably it eventually fades away like a supernova, but we do not know how far it typically builds up first. Perhaps to a violent and self-destructive catastrophe. Perhaps to a more gentle and repeated emission of objects,moving, in a guided rather than a simple ballistic trajectory, away from the star into distant reaches of space, where it may infect other star systems with the same tendency to explode.
The reason we know so little about replication bombs in the universe is that we have seen only one example, and one example of any phenomenon is not enough to base generalizations on. Our one case history is still in progress. It has been under way for between three billion and four billion years, and it has only just reached the threshold of spilling away from the immediate vicinity of the star. The star concerned is Sol, a yellow dwarf star lying toward the edge of our galaxy, in one of the spiral arms. We call it the sun. The explosion actually originated on one of the satellites in close orbit around the sun, but the energy to drive the explosion all comes from the sun. The satellite is, of course, Earth, and the four-billion-year-old explosion, or replication bomb, is called life. We humans are an extremely important manifestation of the replication bomb, because it is through us— through our brains, our symbolic culture and our technology—that the explosion may proceed to the next stage and reverberate through deep space.
As I have said, our replication bomb is, to date, the only one we know of in the universe, but this does not necessarily mean that events of this kind are rarer than supernovas. Admittedly, supernovas have been detected three times as frequently in our galaxy, but then supernovas, because of the immense quantities of energy released, are much easier to see from a long distance. Until a few decades ago, when man-made radio waves started to radiate outward from the planet, our own life explosion would have gone undetected by observers even on quite close planets. Probably the only conspicuous manifestation of our life explosion until recent times would have been the Great Barrier Reef.
A supernova is a gigantic and sudden explosion. The triggering event of any explosion is that some quantity is tipped over a critical value, after which things escalate out of control to produce a result far larger than the original triggering event. The triggering event of a replication bomb is the spontaneous arising of self-replicating yet variable entities. The reason selfreplication is a potentially explosive phenomenon is the same as for any explosion: exponential growth—the more you have, the more you get. Once you have a self-replicating object, you will soon have two. Then each of the two makes a copy of itself and then you have four. Then eight, then sixteen, thirty-two, sixty-four. . . . After a mere thirty generations of this duplication, you will have more than a billion of the duplicating objects. After fifty generations, there will be a thousand million million of them. After two hundred generations, there will be a million million million million million million million million million million. In theory. In practice it could never come to pass, because this is a larger number than there are atoms in the universe. The explosive process of self-copying has got to be limited long before it reaches two hundred generations of unfettered doubling.
We have no direct evidence of the replication event that initiated the proceedings on this planet. We can only infer that it must have happened because of the gathering explosion of which we are a part. We do not know exactly what the original critical event, the initiation of self-replication, looked like, but we can infer what kind of an event it must have been. It began as a chemical event.
Chemistry is a drama that goes on in all stars and on all planets. The players in chemistry are atoms and molecules. Even the rarest of atoms are extremely numerous by the standards of counting to which we are accustomed. Isaac Asimov calculated that the number of atoms of the rare element astatine-215 in the whole of North and South America to a depth of ten miles is “only a trillion.” The fundamental units of chemistry are forever changing partners to produce a shifting but always very large population of larger units—molecules. However numerous they are, molecules of a given type—unlike, say, animals of a given species or Stradivarius violins—are always identical. The atomic dance routines of chemistry lead to some molecules becoming more populous in the world while others become scarcer. A biologist is naturally tempted to describe the molecules that become more numerous in the population as “successful.” But it is not helpful to succumb to this temptation. Success, in the illuminating sense of the word, is a property that arises only at a later stage in our story.
losion? I have said that it was the arising of self-duplicating entities, but equivalently we could call it the origination of the phenomenon of heredity —a process we can label “like begets like.” This is not something molecules ordinarily exhibit. Water molecules, though they swarm in gigantic populations, show nothing approaching true heredity. On the face of it, you might think they do. The population of water molecules (H2O) increases when hydrogen (H) burns with oxygen (O). The population of water molecules decreases when water is split, by electrolysis, into bubbles of hydrogen and oxygen. But although there is a kind of population dynamics of water molecules, there is no heredity. The minimal condition for true heredity would be the existence of at least two distinct kinds of H2O molecule, both of which give rise to (“spawn”) copies of their own kind.
Molecules sometimes come in two mirror varieties. There are two kinds of glucose molecule, which contain identical atoms Tinkertoyed together in an identical way except that the molecules are mirror images. The same is true of other sugar molecules, and lots of other molecules besides, including the all-important amino acids. Perhaps here is an opportunity for “like begets like"—for chemical heredity. Could right-handed molecules spawn right-handed daughter molecules and left-handers spawn southpaw daughter molecules? First, some background information on mirror-image molecules. The phenomenon was first discovered by the great nineteenth-century French scientist Louis Pasteur, who was looking at crystals of tartrate, which is a salt of tartaric acid, an important substance in wine. A crystal is a solid edifice, big enough to be seen with the naked eye and, in some cases, worn around the neck. It is formed when atoms or molecules, all of the same type, pile on top of one another to form a solid. They don’t pile up hugger-mugger but in an orderly geometric array, like guardsmen of identical size and immaculate drill. The molecules that are already part of the crystal constitute a template for the addition of new molecules, which come out of a watery solution and fit it exactly, so the whole crystal grows as a precise, geometric lattice. This is why salt crystals have square facets and diamond crystals are tetrahedral (diamond-shaped). When any shape acts as a template for building another shape like itself, we have an inkling of the possibility of self-replication.
Now, back to Pasteur’s tartrate crystals. Pasteur noticed that when he left a solution of tartrate in water, two different kinds of crystal emerged, identical except that they were mirror images of each other. He laboriously sorted the two kinds of crystal into two separate heaps. When he separately redissolved them, he obtained two different solutions, two kinds of tartrate in solution. Although the two solutions were similar in most respects, Pasteur found that they rotated polarized light in opposite directions. It is this that gives the two kinds of molecule their conventional names of leftand right-handed, since they rotate polarized light anticlockwise and clockwise, respectively. As you would guess, when the two solutions were allowed to crystallize out once more, each produced pure crystals that mirrored the pure crystals of the other.
Mirror-image molecules really are distinct in that, as with left and right shoes, no matter how hard you try, you can’t rotate them so that one can be used as a substitute for the other. Pasteur’s original solution was a mixed population of two kinds of molecules, and the two kinds each insisted on lining up with their own kind when crystallizing out. The existence of two (or more) distinct varieties of an entity is a necessary condition for there to be true heredity, but it is not sufficient. For there to be true heredity among the crystals, left- and right-handed crystals would have to split in half when they reached a critical size and each half serve as a template for growth to full size again. Under these conditions we really would have a growing population of two rival kinds of crystals. We truly might speak of “success” in the population, because—since both types are competing for the same constituent atoms—one type might become more numerous at the expense of the other, by virtue of being “good” at making copies of itself. Unfortunately, the vast majority of known molecules do not have this singular property of heredity.
I say “unfortunately” because chemists, trying for medical purposes to make molecules that are all, say, left-handed, would dearly like to be able to “breed” them. But insofar as molecules act as templates for the formation of other molecules, they normally do so for their mirror image, not for their like-handed form. This makes things difficult, because if you start with a left-handed form you end up with an equal mixture of left- and right-handed molecules. Chemists involved in this field are trying to trick molecules into “breeding” daughter molecules of the same handed-ness. It is a very difficult trick to pull off.
In effect, though it probably didn’t involve handedness, a version of this trick was pulled off naturally and spontaneously four thousand million years ago, when the world was new and the explosion that turned into life and information began. But something more than simple heredity was needed before the explosion could properly get under way. Even if a molecule does show true heredity among left-handed and right-handed forms, any competition between them would not have very interesting consequences, because there are only two kinds. Once the lefthanders, say, had won the competition, that would be the end of the matter. There would be no more progress.
Larger molecules can exhibit handedness at different parts of the molecule. The antibiotic monensin, for instance, has seventeen centers of asymmetry. At every one of these seventeen centers, there is a left-handed and a right-handed form. Two multiplied by itself 17 times is 131, 072, and there are therefore 131, 072 distinct forms of the molecule. If these 131, 072 possessed the property of true heredity, with each one begetting only its own kind, there could be quite a complicated competition, as the most successful members of the set of 131, 072 gradually asserted themselves in successive population censuses. But even this would be a limited kind of heredity, because 131, 072, though a large number, is finite. For a life explosion worthy of the name, heredity is needed but so also is indefinite, open-ended variety.
With monensin, we have reached the end of the road, as far as mirrorimage heredity is concerned. But left-handedness versus right-handedness is not the only kind of difference that might lend itself to hereditary copying. Julius Rebek and his colleagues at the Massachusetts Institute of Technology are chemists who have taken seriously the challenge of producing self-replicating molecules. The variants they exploit are not mirror images. Rebek and colleagues took two small molecules—the detailed names don’t matter, let’s just call them A and B. When A and B are mixed in solution, they join up to form a third compound called—you’ve guessed it—C. Each C molecule acts as a template, or mold. The As and Bs, floating free in solution, find themselves slotting into the mold. One A and one B are jostled into position in the mold, and they thereby find themselves correctly aligned to make a new C, just like the previous one. The Cs don’t stick together to form a crystal but split apart. Both Cs are now available as templates to make new Cs, so the population of Cs grows exponentially.
As described so far, the system doesn’t exhibit true heredity, but mark the sequel. The B molecule comes in a variety of forms, each of which combines with A to make its own version of the C molecule. So we have Cl, C2, C3, and so on. Each of these versions of the C molecule serves as a template for the formation of other Cs of its own type. The population of Cs is therefore heterogeneous. Moreover, the different types of C are not all equally efficient at making daughters. So there is competition between rival versions of C in the population of C molecules. Better yet, “spontaneous mutation” of the C molecule can be induced by ultraviolet radiation. The new mutant type proved to “breed true,” producing daughter molecules just like itself. Satisfyingly, the new variant outcompeted the parent type and rapidly took over the test-tube world in which these protocreatures had their being. The A/B/C complex is not the only set of molecules that behaves in this way. There’s D, E and F, to name just one comparable triplet. Rebek’s group has even been able to make self-replicating hybrids of elements of the A/B/C complex and the D/E/F complex.
The truly self-copying molecules we know in nature—the nucleic acids DNA and RNA—have an altogether richer potential for variation. Whereas a Rebek replicator is a chain with only two links, a DNA molecule is a long chain of indefinite length; each of the hundreds of links in the chain can be any one of four kinds; and when a given stretch of DNA acts as a template for the formation of a new molecule of DNA, each of the four kinds acts as a template for a different particular one of the four. The four units, known as bases, are the compounds adenine, thymine, cytosine and guanine, conventionally referred to as A, T, C and G. A always acts as a template for T, and vice versa. G always acts as a template for C, and vice versa. Any conceivable ordering of A, T, C and G is possible and will be faithfully duplicated. Moreover, since DNA chains are of indefinite length, the range of available variation is effectively infinite. This is a potential recipe for an informational explosion whose reverberations can eventually reach out from the home planet and touch the stars.
The reverberations of our solar system’s replicator explosion have been confined to the home planet for most of the four billion years since it happened. Only in the last million years has a nervous system capable of inventing a radio technology arisen. And only in the last few decades has that nervous system actually developed radio technology. Now, an expanding shell of information-rich radio waves is advancing outward from the planet at the speed of light.
I say “information rich” because there were already plenty of radio waves ricocheting around the cosmos. Stars radiate in the radio frequencies as well as in the frequencies we know as visible light. There is even some background hiss left over from the original big bang that baptized time and the universe. But it is not meaningfully patterned: it is not information-rich. A radioastronomer on a planet orbiting Proxima Centauri would detect the same background hiss as our radioastronomers but would also notice an altogether more complicated pattern of radio waves emanating from the direction of the star Sol. This pattern would not be recognized as a mixture of four-year-old television programs, but it would be recognized as being altogether more patterned and information-rich than the usual background hiss. The Centaurian radioastronomers would report, amid fanfares of excitement, that the star Sol had exploded in the informational equivalent of a supernova (they’d guess, but might not be sure, that it was actually a planet orbiting Sol).
Replication bombs, as we have seen, follow a slower time-course than supernovas. Our own replication bomb has taken a few billion years to reach the radio threshold—the moment when a proportion of the information overflows from the parent world and starts to bathe neighboring star systems with pulses of meaning. We can guess that information explosions, if ours is typical, pass a graded series of thresholds. The radio threshold and, before that, the language threshold come rather late in the career of a replication bomb. Before these was what—on this planet, at least—can be called the nerve-cells threshold, and before that there was the many-cells threshold. Threshold number one, the granddaddy of them all, was the replicator threshold, the triggering event that made the whole explosion possible.
What is so important about replicators? How can it be that the chance arising of a molecule with the seemingly innocuous property of serving as a mold for the synthesis of another one just like itself is the trigger of an explosion whose ultimate reverberations may reach out beyond the planets? As we have seen, part of the power of replicators lies in exponential growth. Replicators exhibit exponential growth in a particularly clear form. A simple example is the so-called chain letter. You receive in the mail a postcard on which is written: “Make six copies of this card and send them to six friends within a week. If you do not do this, a spell will be cast upon you and you will die in horrible agony within a month.” If you are sensible you will throw it away. But a good percentage of people are not sensible; they are vaguely intrigued, or intimidated by the threat, and send six copies of it to other people. Of these six, perhaps two will be persuaded to send it on to six other people. If, on average, one-third of the people who receive the card obey the instructions written on it, the number of cards in circulation will double every week. In theory, this means that the number of cards in circulation after one year will be 2 to the power 52, or about four thousand trillion. Enough postcards to smother every man, woman and child in the world.
Exponential growth, if not checked by lack of resources, always leads to startlingly large-scale results in a surprisingly short time. In practice, resources are limited, and other factors, too, serve to limit exponential growth. In our hypothetical example, individuals will probably start to balk when the same chain letter comes around to them for the second time. In the competition for resources, variants of the replicator may arise that happen to be more efficient at getting themselves duplicated. These more efficient replicators will tend to replace their less efficient rivals. It is important to understand that none of these replicating entities is consciously interested in getting itself duplicated. But it will just happen that the world becomes filled with replicators that are more efficient.
In the case of the chain letter, being efficient may consist in accumulating a better collection of words on the paper. Instead of the somewhat implausible statement that “if you don’t obey the words on the card you will die in horrible agony within a month,” the message might change to “Please, I beg of you, to save your soul and mine, don’t take the risk: if you have the slightest doubt, obey the instructions and send the letter on to six more people.” Such “mutations” can happen again and again, and the result will eventually be a heterogeneous population of messages all in circulation, all descended from the same original ancestor but differing in detailed wording and in the strength and nature of the blandishments they employ. The variants that are more successful will increase in frequency at the expense of less successful rivals. Success is simply synonymous with frequency in circulation. The “St. Jude Letter” is a well-known example of such success; it has traveled around the world a number of times, probably growing in the process. While I was writing this book, I was sent the following version by Dr. Oliver Goodenough, of the University of Vermont, and we wrote a joint paper on it, as a “virus of the mind,” for the journal Nature:
“WITH LOVE ALL THINGS ARE POSSIBLE” This paper has been sent to you for luck. The original is in New England. It has been sent around the world 9 times. The Luck has been sent to you. You will receive good luck within 4 days of receiving this letter pending in turn you send it on. This is no joke. You will receive good luck in the mail. Send no money. Send copies to people you think need good luck. Do not send money cause faith has no price. Do not keep this letter. It must leave your hands within 96 hrs. An A.R.P. officer Joe Elliott received $40, 000, 000. Geo. Welch lost his wife 5 days after this letter. He failed to circulate the letter. However before her death he received $75, 000. Please send copies and see what happens after 4 days. The chain comes from Venezuela and was written by Saul Anthony Degnas, a missionary from S. America. Since that copy must tour the world. You must make 20 copies and send them to friends and associates after a few days you will get a surprise. This is love even if you are not superstitious. Do note the following: Cantonare Dias received this letter in 1903. He asked his Sec’y to make copies and send them out. A few days later he won a lottery of 20 million dollars. Carl Dobbit, an office employee received the letter and forgot it had to leave his hands within 96 hrs. He lost his job. After finding the letter again he made copies and mailed 20. A few days later he got a better job. Dolan Fairchild received the letter and not believing he threw it away. 9 days later he died. In 1987 the letter was received by a young woman in Calif. It was faded and hardly readable. She promised herself she would retype the letter and send it on but, she put it aside to do later. She was plagued with various problems, including expensive car problems. This letter did not leave her hands in 96 hrs. She finally typed the letter as promised and goe a new car. Remember send no money. Do not ignor this—it works.
St. Jude
This ridiculous document has all the hallmarks of having evolved through a number of mutations. There are numerous errors and infelicities, and there are known to be other versions going around. Several significantly different versions have been sent to me from all around the world since our paper was published in Nature. In one of these alternative texts, for instance, the “A.R.P. officer” is an “R.A.F. officer.” The St. Jude letter is well known to the United States Postal Service, who report that it goes back before their official records began and exhibits recurrent epidemic outbreaks.
Note that the catalog of alleged good luck enjoyed by compliers and disasters that have befallen refusers cannot have been written in by the victims/beneficiaries themselves. The beneficiaries’ alleged good fortune did not strike them until after the letter had left their hands. And the victims did not send the letter out. These stories were presumably just invented—as one might independently have guessed from the implausibility of their content. This brings us to the main respect in which chain letters differ from the natural replicators that initiated the life explosion. Chain letters are originally launched by humans, and the changes in their wording arise in the heads of humans. At the inception of the life explosion there were no minds, no creativity and no intention. There was only chemistry.
Nevertheless, once self-replicating chemicals had chanced to arise, there would have been an automatic tendency for more successful variants to increase in frequency at the expense of less successful variants.
As in the case of chain letters, success among chemical replicators is simply synonymous with frequency in circulation. But that is just definition: almost tautology. Success is earned by practical competence, and competence means something concrete and anything but tautological. A successful replicator molecule will be one that, for reasons of detailed chemical technicality, has what it takes to get duplicated. What this means in practice can be almost infinitely variable, even though the nature of the replicators themselves can seem surprisingly uniform.
DNA is so uniform that it consists entirely of variations in sequence of the same four “letters"—A, T, C and G. By comparison, as we have seen in earlier chapters, the means used by DNA sequences to get themselves replicated are bewilderingly variable. They include building more efficient hearts for hippos, springier legs for fleas, aerodynamically more streamlined wings for swifts, more buoyant swim bladders for fish. All the organs and limbs of animals; the roots, leaves and flowers of plants; all eyes and brains and minds, and even fears and hopes, are the tools by which successful DNA sequences lever themselves into the future. The tools themselves are almost infinitely variable, but the recipes for building those tools are, by contrast, ludicrously uniform. Just permutation after permutation of A, T, C and G.
It may not always have been so. We have no evidence that when the information explosion started, the seed code was written in DNA letters. Indeed, the whole DNA/protein-based information technology is so sophisticated—high tech, it has been called by the chemist Graham CairnsSmith—that you can scarcely imagine it arising by luck, without some other self-replicating system as a forerunner. The forerunner might have been RNA; or it might have been something like Julius Rebek’s simple self- replicating molecules; or it might have been something very different: one tantalizing possibility, which I have discussed in detail in The Blind Watchmaker, is Cairns-Smith’s own suggestion (see his Seven Clues to the Origin of Life) of inorganic clay crystals as primordial replicators. We may never know for certain.
What we can do is guess at a general chronology of a life explosion on any planet, anywhere in the universe. The details of what will work must depend on local conditions. The DNA/protein system wouldn’t work in a world of chilled liquid ammonia, but perhaps some other system of heredity and embryology would. Anyway, those are just the kinds of specifics I want to ignore, because I want to concentrate on the planet-independent principles of the general recipe. I’ll go more systematically now through the list of thresholds that any planetary replication bomb can be expected to pass. Some of these are likely to be genuinely universal. Others may be peculiar to our own planet. It may not always be easy to decide which are likely to be universal and which local, and this question is interesting in its own right.
Threshold 1 is, of course, the Replicator Threshold itself: the arising of some kind of self-copying system in which there is at least a rudimentary form of hereditary variation, with occasional random mistakes in copying. The consequence of Threshold l’s being passed is that the planet comes to contain a mixed population, in which variants compete for resources. Resources will be scarce—or will become scarce when the competition hots up. Some variant replicas will turn out to be relatively successful in competing for scarce resources. Others will be relatively unsuccessful. So now we have a basic form of natural selection.
To begin with, success among rival replicators will be judged purely on the direct properties of the replicators themselves—for example, how well their shape fits a template. But now, after many generations of evolution, we move on to Threshold 2, the Phenotype Threshold. Replicators survive not by virtue of their own properties but by virtue of causal effects on something else, which we call the phenotype. On our planet, phenotypes are easily recognized as those parts of animal and plant bodies that genes can influence. That means pretty well all bits of bodies. Think of phenotypes as levers of power by which successful replicators manipulate their way into the next generation. More generally, phenotypes may be defined as consequences of replicators that influence the replicators’ success but are not themselves replicated. For instance, a particular gene in a species of Pacific island snail determines whether the shell coils to the right or to the left. The DNA molecule itself is not right- or left-handed, but its phenotypic consequence is. Left-handed and right-handed shells may not be equally successful at the business of providing the outer protection for snail bodies. Because snail genes ride inside the shells whose shape they help to influence, genes that make successful shells will come to outnumber genes that make unsuccessful shells. Shells, being phenotypes, do not spawn daughter shells. Each shell is made by DNA, and it is DNA that spawns DNA.
DNA sequences influence their phenotypes (like the direction of coiling of shells) via a more or less complicated chain of intermediate events, all subsumed under the general heading of “embryology.” On our planet, the first link in the chain is always the synthesis of a protein molecule. Every detail of the protein molecule is precisely specified, via the famous genetic code, by the ordering of the four kinds of letters in the DNA. But these details are very probably of local significance only. More generally, a planet will come to contain replicators whose consequences (phenotypes) have beneficial effects, by whatever means, on the replicators’ success at getting copied. Once the Phenotype Threshold is crossed, replicators survive by virtue of proxies, their consequences on the world. On our planet, these consequences are usually confined to the body in which the gene physically sits. But this is not necessarily so. The doctrine of the Extended Phenotype (to which I have devoted a whole book with that title) states that the phenotypic levers of power by which replicators engineer their long-term survival do not have to be limited to the replicators’ “own” body. Genes can reach outside particular bodies and influence the world at large, including other bodies.
I don’t know how universal the Phenotype Threshold is likely to be. I suspect that it will have been crossed on all those planets where the life explosion has proceeded beyond a very rudimentary stage. And I suspect that the same is true of the next threshold in my list. This is Threshold 3, the Replicator Team Threshold, which may on some planets be crossed before, or at the same time as, the Phenotype Threshold. In early days, replicators are probably autonomous entities bobbing about with rival naked replicators in the headwaters of the genetic river. But it is a feature of our modern DNA/protein information-technology system on Earth that no gene can work in isolation. The chemical world in which a gene does its work is not the unaided chemistry of the external environment. This, to be sure, forms the background, but it is quite a remote background. The immediate and vitally necessary chemical world in which the DNA replicator has its being is a much smaller, more concentrated bag of chemicals—the cell. In a way, it is misleading to call a cell a bag of chemicals, because many cells have an elaborate internal structure of folded membranes on which, in which, and between which vital chemical reactions go on. The chemical microcosm that is the cell is put together by a consortium of hundreds—in advanced cells, hundreds of thousands—of genes. Each gene contributes to the environment, which they all then exploit in order to survive. The genes work in teams. We saw this from a slightly different angle in chapter 1.
The simplest of autonomous DNA-copying systems on our planet are bacterial cells, and they need at least a couple of hundred genes to make the components they need. Cells that are not bacteria are called eukaryotic cells. Our own cells, and those of all animals, plants, fungi and protozoa, are eukaryotic cells. They typically have tens or hundreds of thousands of genes, all working as a team. As we saw in chapter 2, it now seems probable that the eukaryotic cell itself began as a team of half a dozen or so bacterial cells that clubbed together. But this is a higher-order form of teamwork and is not what I am talking about here. I am talking about the fact that all genes do their work in a chemical environment put together by a consortium of genes in the cell.
Once we have grasped the point about genes working in teams, it is obviously tempting to leap to the assumption that Darwinian selection nowadays chooses among rival teams of genes—to assume that selection has moved up to higher levels of organization. Tempting, but in my view wrong at a profound level. It is much more illuminating to say that Darwinian selection still chooses among rival genes, but the genes that are favored are those that prosper in the presence of the other genes that are simultaneously being favored in one another’s presence. This is the point we met in chapter 1, where we saw that genes sharing the same branch of the digital river tend to become “good companions.”
Perhaps the next major threshold to be crossed as a replication bomb gathers momentum on a planet is the Many-Cells Threshold, and I’ll call this Threshold 4. Any one cell in our life form, as we saw, is a little local sea of chemicals in which a team of genes bathe. Although it contains the whole team, it is made by a subset of the team. Now, cells themselves multiply by splitting in half, with each one growing to full size again. When this happens, all the members of the team of genes are duplicated. If the two cells do not separate fully but remain attached to one another, large edifices can form, with cells playing the role of bricks. The ability to make manycelled edifices may well be important on other worlds as well as our own. When the Many-Cells Threshold has been crossed, phenotypes can’arise whose shapes and functions are appreciated only on a scale hugely greater than the scale of the single cell. An antler or a leaf, an eye’s lens or a snail’s shell—all these shapes are put together by cells, but the cells are not miniature versions of the large shape. Many-celled organs, in other words, do not grow the way crystals do. On our planet, at least, they grow more like buildings, which are not, after all, the shape of overgrown bricks. A hand has a characteristic shape, but it is not made of hand-shaped cells, as it would be if phenotypes grew like crystals. Again like buildings, manycelled organs acquire their characteristic shapes and sizes because layers of cells (bricks) follow rules about when to stop growing. Cells must also, in some sense, know where they sit in relation to other cells. Liver cells behave as if they know that they are liver cells and know, moreover, whether they are on the edge of a lobe or in the middle. How they do this is a difficult question and a much studied one. The answers are probably local to our planet and I shall not consider them further here. I have already touched upon them in chapter 1. Whatever their details, the methods have been perfected by exactly the same general process as all other improvements in life: the nonrandom survival of successful genes judged by their effects—in this case, effects on cell behavior in relation to neighboring cells.
The next major threshold I want to consider, because I suspect that it, too, is probably of more than local planetary significance, is the High-Speed Information-Processing Threshold. On our planet this Threshold 5 is achieved by a special class of cells called neurons, or nerve cells, and we might locally call it the Nervous System Threshold. However it may be achieved on a planet, it is important, because now action can be taken on a timescale much faster than the one that genes, with their chemical levers of power, can achieve directly. Predators can leap at their dinner and prey can dodge for their lives, using muscular and nervous apparatus that acts and reacts at speeds hugely greater than the embryological origami speeds with which the genes put the apparatus together in the first place. Absolute speeds and reaction times may be very different on other planets. But on any planet an important threshold is crossed when the devices built by replicators start to have reaction times orders of magnitude faster than the embryological machinations of the replicators themselves. Whether the instruments will necessarily resemble the objects that we, on this planet, call neurons and muscle cells is less certain. But on those planets where something equivalent to the Nervous System Threshold is passed, important further consequences are likely to follow and the replication bomb will proceed on its outward journey.
Among these consequences may be large aggregations of data-handling units—"brains"—capable of processing complex patterns of data apprehended by “sense organs” and capable of storing records of them in “memory.” A more elaborate and mysterious consequence of crossing the neuron threshold is conscious awareness, and I shall call Threshold 6 the Consciousness Threshold. We don’t know how often this has been achieved on our planet. Some philosophers believe that it is crucially bound up with language, which seems to have been achieved once only, by the bipedal ape species Homo sapiens. Whether or not consciousness requires language, let us anyway recognize the Language Threshold as a major one, Threshold 7, which may or may not be crossed on a planet. The details of language, such as whether it is transmitted by sound or some other physical medium, must be relegated to local significance.
Language, from this point of view, is the networking system by which brains (as they are called on this planet) exchange information with sufficient intimacy to allow the development of a cooperative technology. Cooperative technology, beginning with the imitative development of stone tools and proceeding through the ages of metal-smelting, wheeled vehicles, steam power and now electronics, has many of the attributes of an explosion in its own right, and its initiation therefore deserves a title, the Cooperative Technology Threshold, or Threshold 8. Indeed, it is possible that human culture has fostered a genuinely new replication bomb, with a new kind of self-replicating entity—the meme, as I have called it in The Selfish Gene—proliferating and Darwinizing in a river of culture. There may be a meme bomb now taking off, in parallel to the gene bomb that earlier set up the brain/culture conditions that made the take-off possible. But that, again, is too big a subject for this chapter. I must return to the main theme of the planetary explosion and note that, once the stage of cooperative technology has been reached, it is quite likely that somewhere along the way the power to make an impact outside the home planet will be achieved. Threshold 9, the Radio Threshold, is passed, and it now becomes possible for external observers to notice that a star system has newly exploded as a replication bomb.
The first inkling external observers will get, as we have seen, is likely to be radio waves spilling outward as a by-product of communications within the home planet. Later, the technological heirs of the replication bomb may themselves turn their deliberate attention outward to the stars. Our own halting steps in that direction have included the beaming out into space of messages specifically tailored for alien intelligences. How can you tailor messages for intelligences of whose nature you have no conception? Obviously it is difficult, and quite possibly our efforts have been misconceived.
Most attention has been given to persuading alien observers that we exist at all, rather than sending them messages with substantial content. This task is the same as that faced by my hypothetical Professor Crickson in chapter 1. He rendered the prime numbers into the DNA code, and a parallel policy using radio would be a sensible way to flag our presence to other worlds. Music might seem a better advertisement for our species, and even if the audience lacked ears they might appreciate it in their own way. The famous scientist and writer Lewis Thomas suggested that we broadcast Bach, all of Bach and nothing but Bach, although he feared it might be taken as boasting. But, equally, music might be mistaken, by a sufficiently alien mind, for the rhythmic emanations of a pulsar. Pulsars are stars that give off rhythmic pulses of radio waves at intervals of a few seconds or less. When they were first discovered, by a group of Cambridge radioastronomers in 1967, there was momentary excitement as people wondered whether the signals might be a message from space. But it was soon realized that a more parsimonious explanation was that a small star was rotating extremely fast and sweeping a beam of radio waves around like a lighthouse. To date, no authenticated communications from outside our planet have ever been received.
After radio waves, the only further step we have imagined in the outward progress of our own explosion is physical space travel itself: Threshold 10, the Space Travel Threshold. Science-fiction writers have dreamed of the interstellar proliferation of daughter colonies of humans, or their robotic creations. These daughter colonies could be seen as seedings, or infections, of new pockets of self-replicating information—pockets that may subsequently themselves expand explosively outward again, in satellite replication bombs, broadcasting both genes and memes. If this vision is ever realized, it is perhaps not too irreverent to imagine some future Christopher Marlowe reverting to the imagery of the digital river: “See, see, where life’s flood streams in the firmament!”
We have so far scarcely taken the first step outward. We have been to the moon but, magnificent as this achievement is, the moon, though no calabash, is so local as scarcely to count as traveling, from the point of view of the aliens with whom we might eventually communicate. We have sent a handful of unmanned capsules into deep space, on trajectories that have no visualizable ending. One of these, as a result of inspiration from the visionary American astronomer Carl Sagan, carries a message designed to be deciphered by any alien intelligence who might chance upon it. The message is adorned with a picture of the species that created it, the image of a naked man and woman.
This might seem to bring us full circle, to the ancestral myths with which we began. But this couple is not Adam and Eve, and the message engraved beneath their graceful forms is an altogether more worthy testament to our life explosion than anything in Genesis. In what is designed to be a universally understandable iconic language, the plaque records its own genesis in the third planet of a star whose coordinates in the galaxy are precisely recorded. Our credentials are further established by some iconic representations of fundamental principles of chemistry and mathematics. If the capsule is ever picked up by intelligent beings, they will credit the civilization that produced it with something more than primitive tribal superstition. Across the gulf of space, they will know that there existed, long ago, another life explosion that culminated in a civilization that would have been worth talking to.
Alas, this capsule’s chance of passing within a parsec of another replication bomb is forlornly small. Some commentators see its value as an inspirational one for the population back home. A statue of a naked man and woman, hands raised in a gesture of peace, deliberately sent on an eternal outward journey among the stars, the first exported fruit of the knowledge of our own life explosion—surely the contemplation of this might have some beneficial effects upon our normally parochial little consciousnesses; some echo of the poetic impact of Newton’s statue in Trinity College, Cambridge, upon the admittedly giant consciousness of William Wordsworth:
And from my pillow, looking forth by light Of moon or favouring stars, I could behold The antechapel where the statue stood Of Newton with his prism and silent face, The marble index of a mind for ever Voyaging through strange seas of Thought, alone.